Table of Contents
3-2 Plate Tectonics
Read Chapter 10 in Physical Geology.
As described in Section 10.1, the concept of continental drift was first conceived by Alfred Wegener just over 100 years ago. In this course, we’re not going to focus on the theories that existed before plate tectonics, but it’s worth reading about continental drift in Section 10.2. As described in Section 10.3, a revolution occurred in geological science between the 1930s and the mid-1960s.
Complete Exercise 10.1 (Section 10.2) to help you understand Wegener’s reasoning.
One of the reasons why Wegener’s theory was largely ignored for 50 years is that it relied on a number of properties of the Earth that simply weren’t understood at the time. For example, Wegener thought that continents moved because they were pushed along over the underlying rocky material, but he couldn’t account for the force that might be doing the pushing, and he couldn’t explain how any known force could overcome the massive amount of friction. Although we now know that the asthenosphere is a relatively weak layer along which plates can slide, and also that convection in the mantle provides some of the push, Wegener and his contemporaries did not have any such knowledge.
Section 10.3 provides a summary of some of the important advances in global geology that were made during the first part of the 20th century. Carefully read that section, making sure you understand the following points:
- In the early 1950s, using magnetic orientation data, it was shown that when ancient rocks in Europe were deposited, the magnetic pole positions were different than they are now. First, it was thought that this was the result of “polar wandering,” but later on geologists realized that this phenomenon was more consistent with the concept of moving continents (Figure 10.3.1).
- During the first half of the 20th century, our understanding of the topography of the sea floor increased dramatically. This knowledge led to the discovery of mid-ocean ridges, deep trenches along continental edges, and chains of seamounts (Figure 10.3.4). Although these features were not fully understood at first, we now know that they are respectively related to sea-floor spreading at divergent boundaries, subduction at convergent boundaries, and volcanism where a plate moves slowly over a mantle-plume.
- The application of seismic sounding over wide areas of the sea floor has enabled geologists to map differences in the thickness of sea-floor sediments, and to see that while sediments are thick in most parts of the ocean, they are very thin in areas near to the mid-ocean ridges (Figure 10.3.5). We now know that these differences can be explained by the fact that the sea floor in these areas is very young and not enough time has passed for thick sediments to accumulate.
- In the 1950s, measurements of heat flow through the seafloor showed that a greater than average amount of heat is produced in ridge areas than elsewhere, and that less heat is produced in areas close to the trenches. This phenomenon was interpreted to be the existence of convection within the mantle as is shown in Figure 3-3.
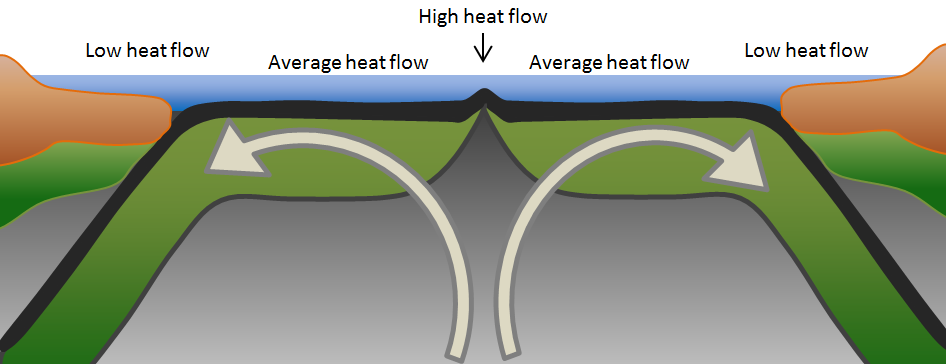
- The development of networks of seismic stations, between the 1930s and the 1950s, enabled a relatively precise positioning (location and depth) of earthquake locations. It was shown that while most significant earthquakes are shallow, they get progressively deeper inshore from the oceanic trenches as is illustrated in Figure 10.3.6.
- In the 1950s and 1960s, the acquisition of magnetic data from the oceans showed a complex pattern of high and low magnetic regions in what was known to be rock of a very consistent basaltic composition (Figure 10.3.7). Although not understood at first, in 1963, Vine, Matthews, and Morley argued that these patterns were a result of new sea-floor crust being formed during times of normal and reversed magnetism.
- Also in 1963, geologists found that the ages of the Hawaiian Islands increased systematically with distance from the still-active volcanoes on the Big Island, which led to the concept that a stationary hot spot (mantle plume) existed beneath the Big Island, and that the Pacific sea-floor crust was moving over top of that source of volcanism (Figure 10.3.9).
- Finally, in 1965, a new type of fault—called a transform fault—was described and explained as being caused by moving plates. Transform faults are common along spreading ridges (Figure 10.3.11), but also are present on land, where they are associated with significant earthquake risks.
In Exercise 10.2, you can see for yourself how the ages of the Hawaiian islands vary with location.
Completing Exercise 10.3 should help you to understand transform faults, and also the process by which the sea floor crust becomes varyingly magnetized.
The theory of plate tectonics finally became widely accepted in the mid-1960s, mostly as a result of the compelling evidence of Vine, Matthews, and Morley, and the work of Tuzo Wilson on hot spots and transform faults. Wilson also played an important role in mapping the boundaries between plates on the Earth’s surface, showing how these plates were moving, and describing the various processes that occur at different types of plate boundaries. Figure 10.4.1 is a modern map of the plates, their directions and rates of motion, and the types of boundaries between them. More than 20 different plates exist, and you should know the names and approximate extents of the seven major ones. A simple way to learn these names and locations is to roughly draw in their boundaries as shown on Figure 3-4. Start with (1) a curvy line down the middle of the Atlantic Ocean. Separate South America from North America (2), and Eurasia from Africa (3). Draw a boundary through the Indian Ocean and separate India and Australia from Asia (4). Draw a line generally around the Pacific Ocean (5), and finally draw a line all the way around Antarctica (6).
Exercise: Without looking at Figure 3-4, draw in the boundaries of the seven major plates on the following map. (If needed, follow the instructions beneath the map.) After you’re done, refer to Figure 10.4.1 in the textbook and add arrows to show the approximate plate motions.
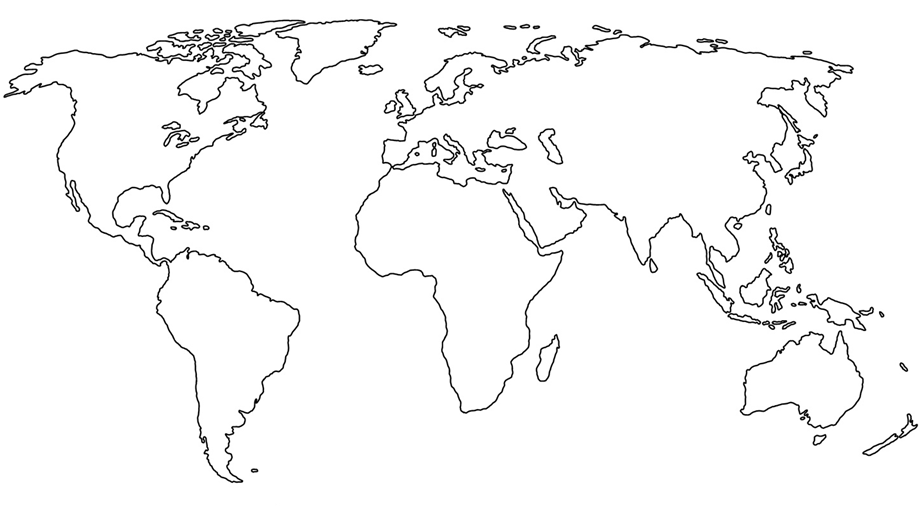
Start with a curvy line down the middle of the Atlantic Ocean. Separate South America from North America, and Eurasia from Africa. Draw a boundary through the Indian Ocean, and separate India and Australia from Asia. Draw a line generally around the Pacific Ocean, and finally draw a line all the way around Antarctica.
To understand plate tectonics, it’s important to know what a plate is. As shown in Figure 10.4.2, tectonic plates are not only comprised of continental or oceanic crust, but also include the underlying lithospheric (“rigid”) part of the mantle. This package of “crust plus lithospheric mantle”—which is about 100 km thick on average—is known as the lithosphere, and it slides across the top of the asthenosphere when a plate moves.
Section 10.4 describes the processes that occur at plate boundaries, starting with divergent (spreading) boundaries where new oceanic crust is made. The key to this process is the convection-related upwelling of hot mantle rock, and the decompression melting of some (about 10%) of that rock within 60 km of the sea floor (Figure 10.4.3). Figure 10.4.4 provides a summary of the types of rocks produced in this environment, which include gabbro at depth, mafic dykes in the middle, and pillow basalts near to the sea floor.
Figure 10.4.5 illustrates the process that is thought to be responsible for the rifting of continents and the formation of new spreading boundaries. Please make sure that you understand this process.
Figures 10.4.6, 10.4.7, and 10.4.8 show two plates moving towards each other at a convergent boundary. Before going any further with this, you need to be aware that tectonic plates never have gaps between them. Convergent boundaries can form within plates, and are most likely to occur at the ocean-continent interface within a plate that includes both oceanic and continental crust.
For example, the North America Plate includes both the continental crust of North America and the oceanic crust of the western Atlantic Ocean. Along the eastern coast of North America, a transition exists between the continental and oceanic crust as depicted in Figure 3-5 (left), which is called a passive margin. Both parts are being pushed to the west by spreading along the mid-Atlantic ridge. At some time in the distant future, the oceanic and continental lithospheres could separate, and subduction could begin along this boundary (Figure 3-5 right). This is likely to be due to the accumulation of sediment along the boundary, as is described in more detail towards the end of Section 10.4.
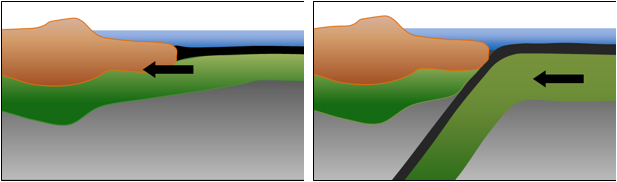
In Section 10.4, read carefully through the material on convergent boundaries. Some of the important points are that water from the subducting crust contributes to melting in the overlying hot mantle rock, and that the compressive stress of convergence leads to faulting and deformation, not only in the continental crust, as is shown in Figure 10.4.7, but also in the oceanic crust on both sides of the boundary.
As already mentioned, most transform faults exist at spreading ridges where they form the boundaries between offset ridge segments. They also form the boundaries between plates, as is illustrated in Figure 3-6. In this figure, the two plates have been highlighted in different colours, and you can see how the ridge segments (double white lines) form the plate boundary at some locations, whereas the transform faults (red lines) form the plate boundary at other locations. The white lines that extend out on either side of the ridge segments are called fracture zones. These are not plate boundaries, and provided that the rates of spreading on the various ridge segments are similar, no relative motion (i.e., no faulting) occurs along these lines.
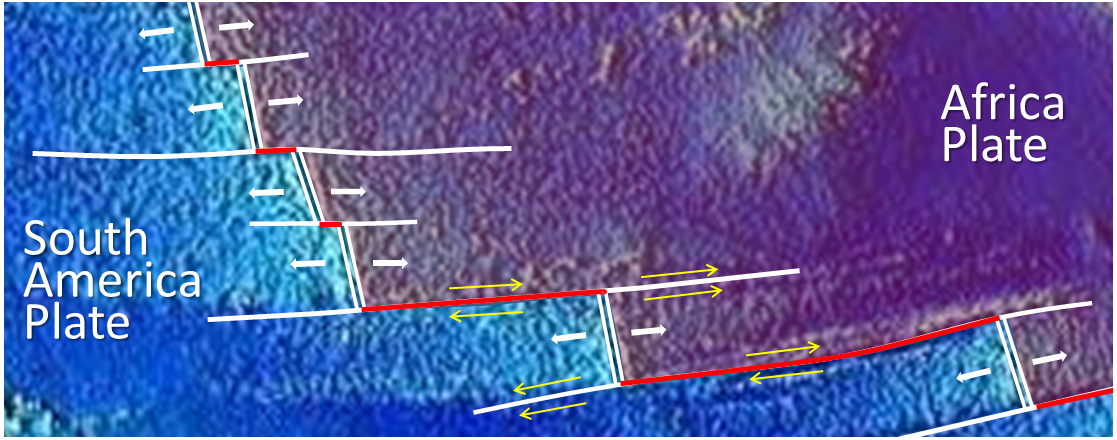
In some cases, transform boundaries cut across continents, for example, the San Andreas Fault in California. All transform faults are characterized by motion that is essentially horizontal—the two plates moved side-by-side past each other.
Completing Exercise 10.4 will contribute to your understanding of transform boundaries.
The remaining part of Section 10.4 includes a summary of some relatively recent and near-future expected changes in the Earth’s tectonic plate configuration. It also includes a discussion of the Wilson cycle and a more complete description of how a passive ocean-continent margin can change into a subduction boundary (Figure 10.4.12).
Test your recollection of plate names, and your understanding of plate boundaries, by completing Exercise 10.5.
Section 10.5 includes a brief discussion of the mechanisms for plate motion. Although we’ve said all along that convection in the mantle is the critical driver of plate tectonics, some related phenomena exist—including the gravitational push away from the elevated ridge areas (ridge push) and the gravitational pull of subducting oceanic crust (slab pull)—that contribute to plate motion. The three processes essential to the movement of plates are summarized in Figure 10.5.2.
Before moving on to Section 3-3 of this unit, on earthquakes, answer the review questions at the end of Chapter 10.