Table of Contents
1-3 Intrusive Igneous Rocks
Read Chapter 3 in Physical Geology.
The first part of Chapter 3 in your textbook includes a description of the rock cycle, which is a simple diagram created to explain the relationships amongst the three main rock types: igneous (rock cooled from magma), sedimentary (rock made up of fragments or dissolved ions derived from existing rock), and metamorphic (rock formed when either sedimentary or metamorphic rocks are heated beyond the stability of one or more of their minerals and changed). In the rock cycle diagram (Figure 3.1.1 in your textbook), two of the important processes are uplift and burial. Uplift is typically achieved by plate tectonic processes, mostly the convergence of plates (moving towards each other) that results in collisions between continents and the formation of mountain ranges. During that process, some rocks get pushed up to form mountain peaks, whereas other rocks get buried deep beneath these mountains. Burial also is achieved in sedimentary environments by the continual process of sedimentation and the very slow build-up of hundreds or thousands of metres of sediments on top of older sediments.
Completing Exercise 3.1 will give you some practice in using the rock cycle, and should also help you to understand the amount of time required for some of these processes to occur.
Section 3.2 describes magma and its formation. Magma is a mixture of elements held at such a high temperature that none of them is able to combine with other elements to form minerals, and so the material is liquid. Temperatures in the order of 800 to 1300˚C are typical of magmas in the upper part of the crust. Almost all magmas are dominated by the elements shown in Figure 3.2.1, but the proportions of these can vary quite widely.
All the magma in the Earth’s crust and mantle forms by the process of a partial melting of existing rock as is illustrated in Figure 3.2.2. As conditions slowly change within the crust or mantle, some of the components of the rock become unstable and start to melt. This partial melting creates a magma that has a different composition than rock—magma always has a higher proportion of silicon (and less iron and magnesium) than the rock that is being melted.
It’s worth repeating here what is stated in the textbook—that contrary to what you might naturally think, the partial melting of rock within the crust and mantle does not normally occur because the rock is heated; instead, melting is typically caused by a decrease in pressure (decompression melting) or the addition of a substance (typically water) that has an effect of reducing the melting temperature of the rock (flux melting) that is already hot and close to its melting point. These processes are illustrated in Figure 3.2.3.
Decompression melting is more easily understood than flux melting. Imagine a hot substance that is being kept under pressure. Although the heat makes the atoms move around rapidly to the point where their bonds can break, the pressure contains that effect, keeping them together. When the pressure is reduced, containment is reduced, and some of the substance can melt. Flux melting is based on the principle that a mixture of substances—for example, rock plus water—has a lower melting point than just the rock.
Plate tectonics can account for both of these processes as illustrated in Figure 3.2.4. Two important processes result in upward movement of mantle rock: convection and mantle plumes. As very hot, but still solid, rock moves towards surface, the pressure to which it has been subject decreases immediately, but its temperature decreases very slowly, and the drop in pressure leads to melting. At a subduction zone, oceanic crust is pushed (subducted) down into the mantle. Water from that crust migrates upward into the hot mantle rock, which lowers its melting point and leads to partial melting. This process is shown in a little more detail in Figure 1-5.
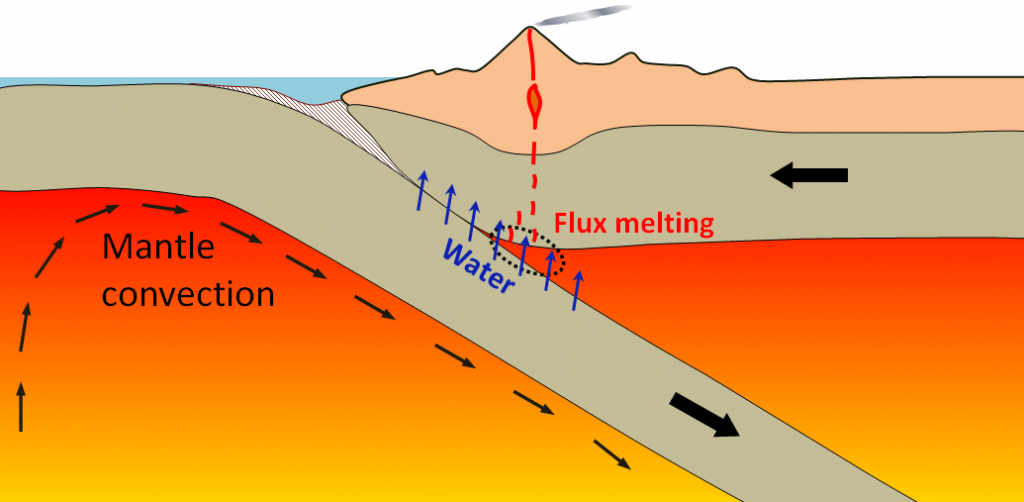
Although we stated earlier that all of the atoms in liquid magma are uncombined, this interpretation is not exactly correct. Oxygen and silicon have a very strong affinity for each other, and even while a cooling magma is still very hot, they will start to combine to form silica tetrahedra, and those tetrahedra will start to join together into chains known as polymers. Silica polymers make the magma more viscous (less runny), and the more silica that is in the magma, the more viscous it will be as it starts to cool.
You can experience for yourself how this works by doing Exercise 3.2.
Magma within the upper mantle and crust tends to move slowly towards the surface because it is lighter than the surrounding rock, and eventually this movement reduces its temperature, which leads to crystallization. Like melting, crystallization is a discontinuous and complicated process because although hot magma may look like a simple liquid—such as liquid candle wax or molten steel—it is very different. As it cools, crystals of different minerals start to form in a generally predictable sequence, which was first described in any detail by Norman Bowen over 100 years ago (see Figure 3.3.1). The sequence of crystallization depends somewhat on the original composition, but generally it starts with olivine or pyroxene and plagioclase feldspar.
Please read the section on Bowen’s reaction series in Section 3.3 carefully, since it is a complicated process that has important implications for the textures and mineral compositions of igneous rocks. In the context of this process, make sure you understand the significance of the word reaction.
Magma composition can vary quite widely as illustrated is in Figure 3.3.4, with the most significant variations being in the proportions of silica, iron, magnesium, and calcium. Magmas (and the rocks that form from them) are classified as felsic if they have more than 65% silica (as SiO2), intermediate if they have between 55 and 65% silica, and mafic if they have between 45 and 55% silica. Mafic magmas have much more iron, magnesium, and calcium—and less sodium and potassium—than felsic magmas.
Completing Exercise 3.3 will help you to understand the differences between mafic, intermediate, and felsic magmas.
Figure 3.3.5 provides a simplified classification of igneous rocks by composition. Using the same three categories—mafic, intermediate and felsic—we can classify coarse-crystalline intrusive igneous rocks as gabbro, diorite, and granite respectively, and their fine-crystalline volcanic counterparts as basalt, andesite, and rhyolite. By the time you finish this course, you should be very familiar with these six important igneous rock types, and their characteristics.
In many cases, the magma that forms the igneous rocks we see at surface has spent a long time (tens of thousands to millions of years) in a magma chamber within the crust. Some of the processes that take place within a magma chamber are illustrated in Figure 3.3.6. Since temperature increases with depth in the Earth, we can expect that a magma chamber that extends over a few kilometres from bottom to top will be significantly cooler (by as much as 100˚C) at the top than the bottom. Fractional crystallization is the process during which crystals form in the upper part of the magma chamber and then slowly sink towards the bottom. Since these early-forming crystals, like olivine or pyroxene, tend to be iron- and magnesium-rich, iron and magnesium are removed from the upper part of the chamber and transported to the bottom, where these crystals may re-melt due to the greater heat.
Another process that is not shown in Figure 3.3.6 is the partial melting of the rock surrounding the magma chamber. Since partial melting favours the melting of silica-rich minerals, such as quartz and feldspar, it typically results in an overall increase in the silica content of the magma.
When a magma cools in two stages—first slow and then much faster—the resulting rock can have a special texture known as porphyritic in which crystals of one or more minerals are much larger than those of the rest of the minerals in the rock (see Figure 1-6 and Figure 3.3.7 in the textbook).
Complete Exercise 3.4 to gain a better understanding of the process of the formation of porphyritic textures.
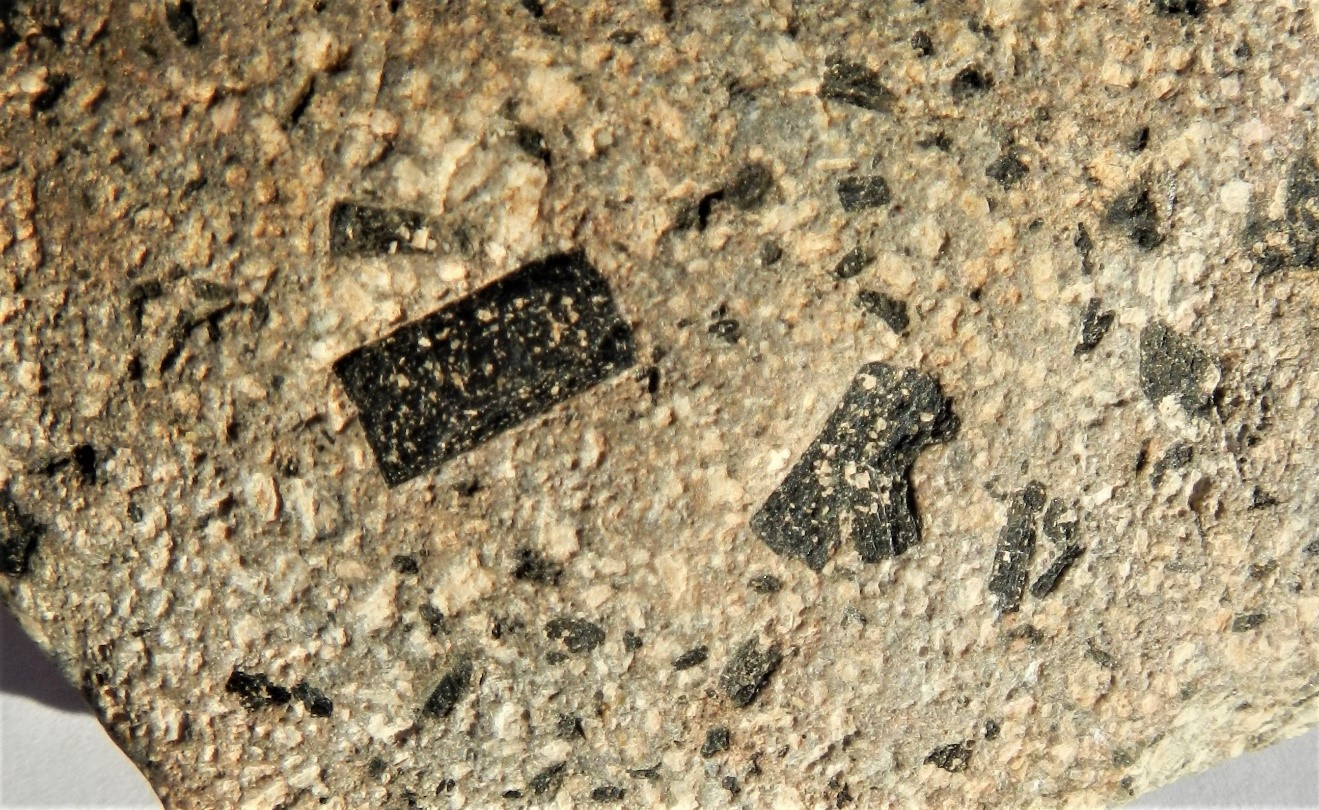
As described in Section 3.4 in your textbook, igneous rocks can be classified according to the proportions of different minerals they contain. In this case, we are talking only about silicate minerals because they make up more than 99% of most igneous rocks. Figure 3.4.1, one tool for doing these kinds of classifications, shows ferromagnesian silicates in shades of green, and non-ferromagnesian silicates in shades of pink, white, and grey.
Some students have difficulty using this diagram (Figure 3.4.1), so it is strongly recommended that you practise with it by completing Exercise 3.5. To help you get started, note that a rock represented by the dotted black line separating “FELSIC” from “INTERMEDIATE” has the following composition: quartz: ~28%, plagioclase feldspar: ~52% (because it extends from 28 to 80%), and biotite/amphibole: ~20% (because it extends from 80 to 100%).
Note for registered students: In the field exercise of Assignment 1, you are asked to find an intrusive igneous rock somewhere in your region, and to describe its texture and estimate the proportions of the different minerals within it. This can be tricky, but you can get some practice with it by doing Exercise 3.6 in which you are asked to estimate only the proportion of the dark (ferromagnesian) minerals, although the same principle can be applied to other minerals.
Igneous rock textures are determined by the rate and conditions of cooling. Rocks that cool slowly have a phaneritic texture with visible crystals (Figure 1-7, left). If the magma is rich in water during crystallization, the crystals can grow very large, which creates a pegmatitic texture as shown in Figure 3.4.4 in the textbook. When magma cools quickly—typically during a volcanic eruption—the crystals are too small to see (aphanitic texture—Figure 1-7, centre). Some volcanic eruptions are explosive, producing volcanic rock fragments of varying sizes embedded in volcanic ash (pyroclastic texture—Figure 1-7, right).
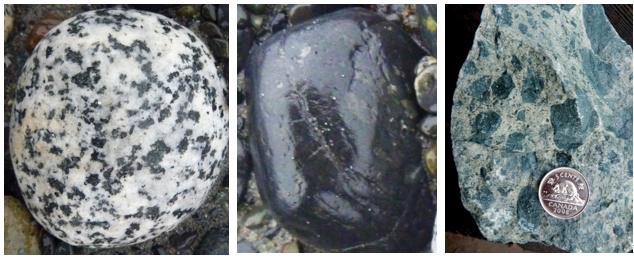
Intrusive igneous rocks are so named because they “intrude” into previously existing rock (known as country rock) and form bodies (known as plutons) with a variety of shapes, sizes, and orientations with respect to the fabric of the country rock.
In Section 3.5, read about dykes, sills, stocks, and batholiths, and then do Exercise 3.7 to test your understanding. Some of these identifications are tricky, so check in the course textbook to see if your answers are correct.
Please complete the questions for review at the end of Chapter 3.