Table of Contents
1-2 Minerals
Read Chapter 2 in Physical Geology. To understand minerals, we need to know something about the bonding between atoms, and to understand that, we need to go back to what an atom looks like, and especially how electrons are arranged around a nucleus. Section 2.1 in your textbook provides a description. If this is review for you, then so much the better. If it isn’t, please read carefully.
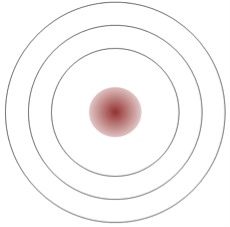
OK, now that you’ve done a careful reading, (and without referring back to Table 2.2 in the textbook), consider element number 5: boron (B). How many electrons does it have in the first shell, and how many in the second? How about element number 9: fluorine (F)?
In Section 2.2, we look at ionic and covalent bonding. In an ionic bond, electron configuration issues are satisfied by electron transfer. In a covalent bond, electron configuration issues are satisfied by the sharing of electrons.
It’s important to complete Exercise 2.1, and then check your answers in your course textbook.
Section 2.2 also has a discussion on the bonding that occurs within a silica tetrahedron. This bonding is critical because so much of the Earth’s crust and mantle are made primarily of silicate minerals, all of which include silica tetrahedra bonded in various ways.
The last part of Section 2.2 is a discussion of mineral lattices, which includes a photo of some halite crystals on the left, and broken halite crystals on the right (see Figure 2.2.6). To refresh your memory, the lattice for halite is shown in Figure 1-2. All of the angles between the atoms are right angles, which helps to explain why halite grows as perfect cubes and also why it breaks along those same planes (known as cleavage planes). Note that halite is somewhat unique in this respect. In most minerals, the cleavage planes are not parallel to the crystal faces.
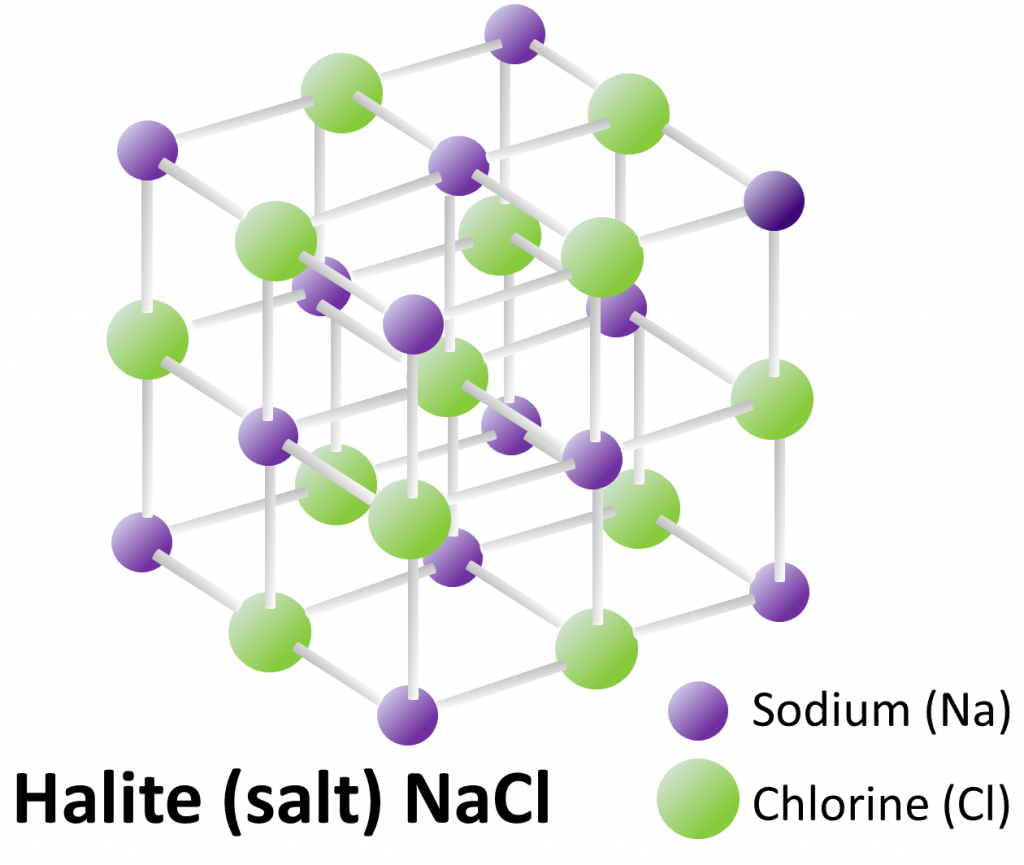
Thousands of different minerals exist, so it’s important to be able to classify them into groups, as described in Section 2.3.
To make sure that you understand the rules for classification of minerals into groups, please complete Exercise 2.2.
The important silicate mineral group is described in Section 2.4. As already noted, all silicate minerals include silica tetrahedra that are arranged in a variety of ways. In some minerals, such as olivine or garnet, the tetrahedra are isolated from each other and bonded to other elements, such as iron or magnesium. In other silicates, the tetrahedra are bonded to each other in a variety of configurations, and also bonded to other elements.
Please do Exercise 2.3 to better understand what a tetrahedron looks like. A copy of the figure that you need to cut out is included as Figure 1-3, so just print the page. Once you’ve made a tetrahedron, you need to visualize that there is an oxygen atom at each of the four corners, and a silicon atom in the middle (see Figure 1-4 below).
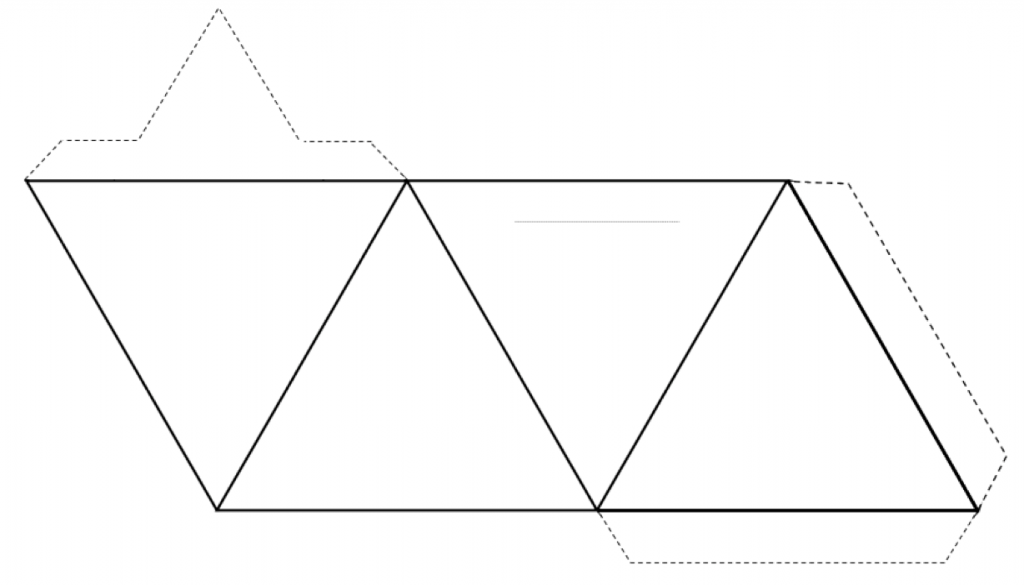
Table 2.6 in your textbook is a summary of the different arrangements of tetrahedra in silicate minerals, from isolated (e.g., olivine or garnet) to pairs (epidote), rings (tourmaline), single chains (pyroxene), double chains (amphibole), sheets (mica), and 3-dimensional frameworks (feldspar and quartz). Read carefully about these structures, and don’t underestimate the importance of the table of ion sizes (i.e., radii) provided in Figure 2.4.2. These sizes play important roles in determining which elements will fit into which minerals, and also which elements might substitute for each other in certain elements.
Pay special attention to the discussion of feldspars, because they are the most common minerals in the Earth’s crust. There are two main types of feldspars, as illustrated in Figure 2.4.6. Potassium feldspar includes potassium (K) along with aluminum (Al) and silica (Si), whereas the plagioclase feldspars can contain sodium (Na) and calcium (Ca) in varying proportions, along with aluminum and silica. Plagioclase has so many different types because Na and Ca are virtually identical in size (Figure 2.4.2), and so can easily substitute for each other in the feldspar mineral structure. Potassium, on the other hand, is too large to fit into the plagioclase structure.
The nature of silica tetrahedra bonding in silicates not only is important for determining their structure, but also has implications for their chemistry. As described in the textbook, an isolated tetrahedron has 4 oxygens and 1 silicon, and because oxygen ions have a charge of -2 and silicon ions a charge of +4, the tetrahedron has an overall charge of -4 (see Figure 1-4). This negative charge has to be balanced by cations such as iron and magnesium, but when tetrahedra are bonded together (in chains or sheets, for example), the oxygens in the bonded corners are shared, which means that some of the oxygens are removed from the structure, and the ratio of oxygens to silicons decreases, and fewer cations are needed to create a charge balance.
Completing Exercise 2.4 will help you to understand the concepts of bonding and oxygen sharing in silicate minerals.
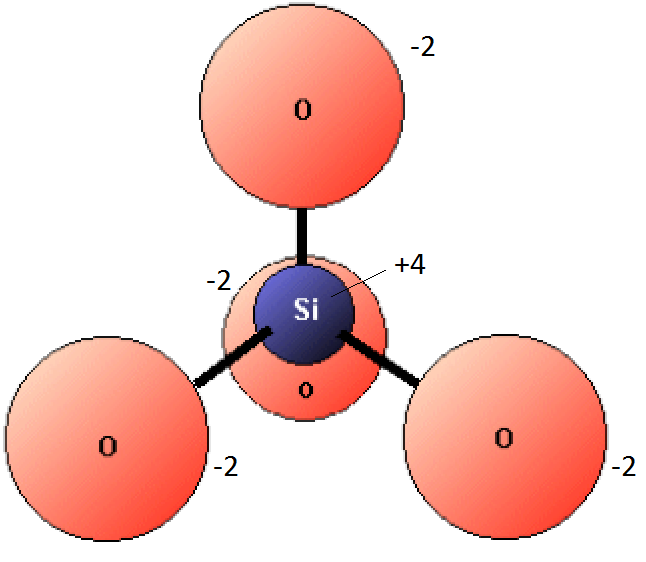
Silicate minerals also are grouped according to whether they contain iron and/or magnesium. Those that do—such as olivine, pyroxene, amphibole, and biotite mica—are called ferromagnesian silicates. They tend to be both darker and heavier than the non-ferromagnesian silicates, which include muscovite mica, the feldspars, and quartz.
Completing Exercise 2.5 will help you to understand the difference between ferromagnesian and non-ferromagnesian silicates. Some of these are tricky, so check your answers in the course textbook.
Section 2.5 provides a summary of the various ways that minerals can form. The vast majority crystallize during the cooling of magma, but some form in other ways. Note for registered students: In one of the exercises in Assignment 1, you’ll be asked to create some mineral crystals through precipitation from aqueous solution. If you wish, you may get started on that now.
Note for registered students: In one of the exercises in Assignment 1, you’ll be asked to create some mineral crystals through precipitation from aqueous solution. If you wish, you may get started on that now.
To identify one mineral from another, geologists (and geology students) rely on a series of physical properties that are relatively easy to determine by testing a small sample of a mineral. These properties include colour, streak (the colour of a powder made from the mineral), hardness, habit (the way the mineral grows as crystals), cleavage and fracture (the way the mineral breaks), density, and a few others.
One thing to bear in mind is that these properties apply to minerals, but not to rocks. So, if you wanted to test for one of these properties in a rock sample that has visible mineral crystals or grains in it, you have to be careful to actually test the specific mineral of interest.
Please do the questions at the end of Chapter 2 in your textbook. Again, the answers to the questions are available in Appendix 2.