Table of Contents
3-1 Earth’s Interior
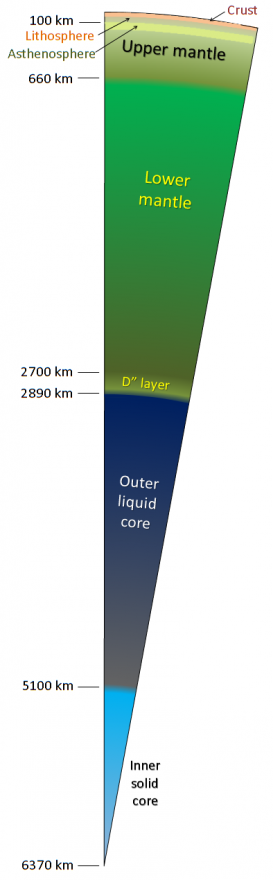
Read Chapter 9 in Physical Geology.
The diagram to the left (Figure 3-1), which is similar to Figure 9.0.2 in the text, shows the main features of the Earth’s interior. The outermost part is the crust. It is 30 to 40 km thick in most areas, making it equivalent in thickness to the skin of an apple. The next part, and the greatest in volume by far, is the mantle, which makes up 83% of the volume of the Earth. The mantle consists of ferromagnesian silicate minerals and can be divided into five distinct layers: the rigid lithosphere, the nearly-liquid asthenosphere, upper and lower mantle (both solid, but able to flow when subjected to a slow force), and the D” layer, which is also nearly liquid. The overall compositions of these different parts of the mantle are not significantly different, but they have differing physical properties of mineralogy (e.g., although the upper and lower mantle are made of different minerals, they have the same compositions overall). Although the core-mantle boundary (CMB) is approximately halfway to the centre of the Earth, the volume of the core (16% of the Earth’s volume) is much less than that of the mantle. The core is primarily made of iron. The outer core is liquid, and the inner core, although hotter, is solid because it is under such intense pressure. As described in Section 9.1, our main source of information about the Earth’s interior is the seismic data from the vast array of seismometers installed at every corner of the planet. Every time a significant earthquake occurs, seismic waves spread through the Earth and are detected at thousands of different locations..
Figure 3-1. Main features of the Earth’s interior. © Steven Earle. Used with permission.
All of the detected waves travel though the different parts of the crust, mantle, and core, and their velocities and behaviour provide information about the nature of those various regions of the Earth’s interior. Before we go any further, it’s important to understand that the waves that pass through the Earth are known as body waves, and that they come in two types: P waves and S waves. The differences between the two are described in the textbook. The critical things to know are that P waves travel faster than S waves, that both types of waves travel faster in hard and strong materials than in soft and weak materials, and that while P waves will pass through a liquid, S waves will not.
The seismic wave velocity diagrams of Figure 9.1.4—which show us how the properties of the materials within the Earth change—are based on information from thousands of earthquakes collected at hundreds of seismic stations. The most dramatic changes occur at the core-mantle boundary where the S waves stop altogether, and the P waves slow dramatically because the outer core is liquid. However, other more subtle changes also happen within the different layers of the mantle. For example, all waves are slowed within the zone called the asthenosphere because the rock is partially molten, and therefore softer and weaker than the rest of the mantle.
Completing Exercise 9.1 will give you some idea of the rate at which P waves travel through the crust.
Section 9.1 in the textbook includes a list of the important behaviours of seismic waves as they pass through the Earth. This is worth repeating here, and while reading this list make sure that you can point out these features in Figures 9.1.4, 9.1.5, and 9.1.6:
- Velocities are greater in the mantle than in the crust, which explains the Mohorovičić discontinuity illustrated in Figure 9.1.5.
- Velocities generally increase with pressure, and therefore with depth, which accounts for the curved-outward pattern of the waves within the Earth as illustrated in Figure 9.1.6.
- Velocities slow in the area between a 100 km and 250 km depth (called the low-velocity zone, which is equivalent to the asthenosphere) as shown in Figure 9.1.4.
- Velocities increase dramatically at 660 km depth because of a mineralogical transition.
- Velocities slow in the region just above the core-mantle boundary (known as the D” layer or ultra-low-velocity zone).
- S waves do not pass through the outer part of the core, and P wave velocities are dramatically slowed at this boundary.
- P-wave velocities increase at the boundary between the liquid outer core and the solid inner core.
Complete Exercise 9.2 to help with your understanding of the nature of seismic wave propagation through the Earth.
Section 9.2 describes the pattern of temperature changes within the Earth. As everyone knows, the further down you go into the Earth, the hotter it gets, but the rate of temperature increase is not consistent. The temperature increases quickly in the crust and the uppermost part of the mantle, and then less quickly in the mantle (Figure 9.2.1). This change is attributed to mixing within the mantle. Like a pot of very thick soup on the stove, the mantle is being heated from below (by the hot core). If mixing was not occurring in the soup pot, the bottom part would get very hot and probably burn, while the top would remain relatively cool. This situation would be reflected by a steep temperature gradient (a relatively flat line in Figure 9.2.1). If the soup was being stirred continuously, the temperature would be the same throughout (a vertical line in Figure 9.2.1). The mantle is somewhere between these end points because it is being mixed slowly—not by someone “stirring the pot,” but by convection—so the temperature difference between the bottom and the top is not as great as it would be otherwise. The convection occurring within the mantle (illustrated in Figure 9.2.2) is a critical component of plate tectonics, which we’ll talk more about later in this unit.
As described at the end of Section 9.2, the sources of heat in the Earth’s interior are mainly leftover heat from the collisions that formed the Earth, and the heat created by natural radioactive decay. The amount of available heat energy is gradually decreasing as the Earth is cooling, but unlike some smaller bodies (Mars and the Moon, for example), enough heat remains to maintain a liquid core, to drive mantle convection and plate tectonics, and to produce volcanism.
As just mentioned, the Moon and Mars no longer have liquid cores. Go briefly back to Exercise 9.2 and think about what that means for the S wave shadow zones in these bodies.
Section 9.3 describes the origins and behaviour of the Earth’s magnetic field. As is summarized in this section, the Earth has a magnetic field because the outer part of the iron-rich core is still liquid and because that material is moving convectively. This field is similar to the typical dipole (two poles, north and south) of a bar magnet as illustrated in Figure 9.3.1, which means that at the Earth’s surface, the orientation of the magnetic field will vary depending on latitude and longitude. As we’ll see later, this magnetic field has very significant implications for how geologists came to understand plate tectonics.
You can increase your understanding of the orientations of the magnetic field lines by completing Exercise 9.3.
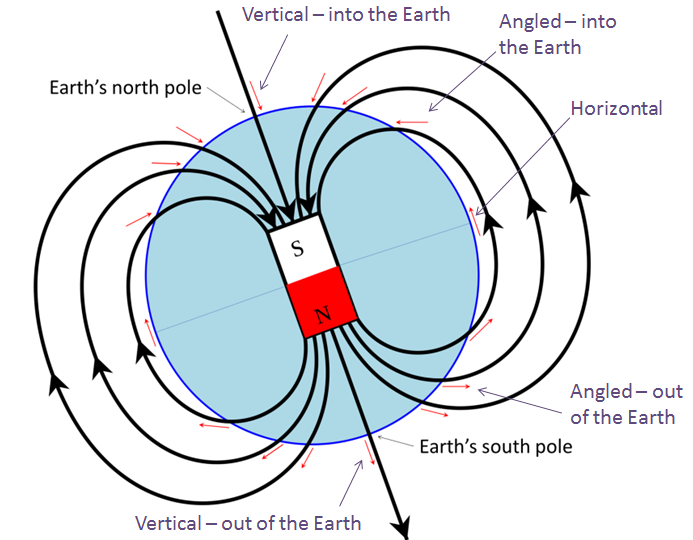
The Earth’s magnetic field is not static. Rather, it varies in intensity, and periodically (on time scales of thousands of years) fades away to almost nothing and then re-establishes itself, sometimes with the opposite polarity (the north arrow of a compass would point to the South Pole). We now understand the chronology of magnetic field reversals, which also has significant implications for how geologists came to understand plate tectonics.
The final section of Chapter 9 includes an overview of isostasy, which we’ve already discussed in the context of metamorphism. The key factor is that the mantle is plastic; it flows or deforms in response to a slowly-applied stress. When a change occurs to the mass of part of the crust—because of mountain building, erosion, glaciation, or deglaciation—the mantle responds by allowing the crust to sink deeper or float higher than before. This process is illustrated in Figures 9.4.2 and 9.4.3.
The crust beneath the oceans is compositionally different than that of the continents. Oceanic crust is heavier and sinks lower on the mantle—which is why oceans are below sea level while most parts of the continents extend above sea level.
You can see how this works by completing Exercise 9.4. The differences in the densities of oceanic and continental crust play an important role in how the Earth’s plates interact with each other.
Complete the review questions at the end of Chapter 9, and check your answers in Appendix 2 in the textbook.